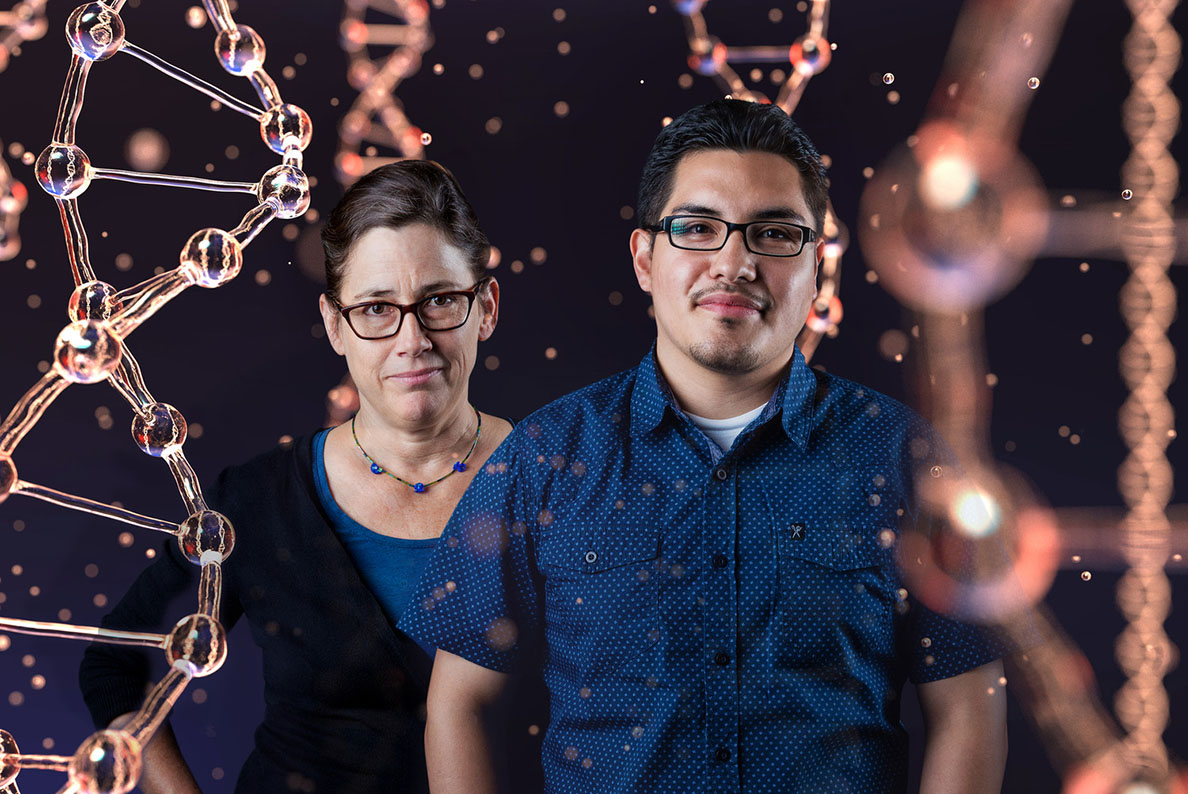
The Real Reason You Need to Know About CRISPR
- BY KRISTA DOSSETTI
- PHOTOGRAPHY BY GARVIN TSO
- August 10, 2017
The sci-fi-tinged talk of a distant reality has never been nearer. If you haven’t yet heard of CRISPR, you’re late to the party for the future of gene-editing technology. Trouble with mosquitoes? No longer. Hungry for mildew-resistant crops? Problem solved. The elusive cure for cancer? Check. The promises of CRISPR are near endless, and equally fraught with ethical questions (Designer babies? Tampering with evolution?), but if you follow popular science, you’ve likely heard the revolutionary technique hailed as everything from the future of mankind to a weapon of mass destruction.
Notwithstanding moral and legal quandaries about how the gene-editing technology can be used, there’s a glaring problem. What CRISPR means for the future of science, particularly medical advances, begs a question that is central to many discussions of discovery: How do we bridge the gap between what is possible and what is practical? Between what we can do and what we should do? Between wild fantasy and a successful treatment?
The answer is evolving at Cal State East Bay, where students in a graduate-level course are being trained in CRISPR — an experience master’s degree student Claudina Kwok says wasn’t possible to come by during her undergraduate years at a UC.
“It was all class sizes of 200-300 people and lab classes were taught by a teacher’s assistant,” Kwok says. “I definitely got more hands-on experience and more lab experience at Cal State East Bay. It’s important to have small classes and direct access to a professor who makes sure you understand every step in learning a technique like this. The ability to use CRISPR opens up a lot of opportunities for my future.”
Kwok is now working on her master’s thesis (an early stage study using CRISPR to assess the impact of gene modification on alcoholic behaviors), and is one of dozens of students who have taken Professor of Biological Sciences Maria Gallegos’ Advanced Molecular Techniques class.
Gallegos, who has been following the work of leading researchers in the United States, including CRISPR pioneers Jennifer Doudna and Feng Zhang (see The Battle for CRISPR), returned to Cal State East Bay after a 2014 sabbatical “itching” to use the technique in her own research, she says. And, as long as she was learning about CRISPR, she felt she should teach it, too.
“It’s really important to me that the graduate students come out of the Biotechnology Certificate and master’s program with useful skills and knowledge,” Gallegos says. “A deep understanding of CRISPR on both the practical and theoretical level is really desirable in biotech, not because the industry wants to create designer babies, but because it’s interested in understanding disease and developing treatments — and that’s where most of our students hope to build careers. CRISPR is so new that very few universities are performing it in the classroom with their students. I thought it would be a perfect vehicle for teaching advanced molecular techniques.”
A STAR IS BORN
CRISPR, short for Clustered Regularly Interspaced Short Palindromic Repeats, was first described in the 1990s as a short sequence of DNA that repeats itself along a portion of the genomes found in bacteria. These repeats are interrupted by “spacers” that function like a warehouse of viral immunity — each time there is exposure to a new virus, the memory of it is recorded in the spacers, so that future threats can be recognized and eliminated.
“But the cutting alone isn’t what makes CRISPR revolutionary — it’s the ability to add new information into the genome through what’s called a ‘knock in.’”
As scientists worked to understand the seemingly random content in the spacers, they came across a gene that produces an enzyme (Cas9) with an especially useful talent: to cut DNA at a precise location.
But the cutting alone, Gallegos explains, isn’t what makes CRISPR revolutionary — it’s the ability to add new information into the genome through what’s called a “knock in.”
“When a cell’s DNA is cut, it naturally works hard to repair the damage in one of two possible ways,” she says. “The first way is a messy soldering of the two cut ends that typically leaves a scar (called a mutation). This produces a ‘knock out’ and can help scientists learn how an organism functions in the absence of a particular gene. What we want to do in my lab are called ‘knock-ins,’ which exploit the cell’s natural ability to use matching DNA to try and fix the cut made by CRISPR. We create the matching DNA in the lab to include the component we want to ‘knock in.’”
It may sound complicated, but scientists are hailing CRISPR as incredibly simple — easy even. Although previous breakthroughs in gene editing foresaw the same victories as CRISPR, the new technique promises to whittle down a process that once took months or even years to weeks and days, and could be used to fix genes such as those that cause cystic fibrosis or allow mosquitoes to spread malaria, among innumerable other possibilities.
Yet Gallegos, whose focus is in neurobiology, cautions that some important foundational work needs to happen before those wins can play out en masse.
“Humans are complex multicellular creatures,” she says. “Sure, there are some conditions like the blood-based disease sickle-cell anemia that I believe CRISPR could be used to treat very soon, but in general, we need a more comprehensive picture of how genes function within organisms and how species function within ecosystems before we start fiddling with either. We need to think long and hard about the implications we’re creating for future generations. At this stage, the promise of CRISPR is in its incredible ease of use, and capacity to facilitate research and a better understanding of how genes work.”
WHAT WE DON’T KNOW
- elegans,a microscopic, transparent round worm, has long been the object of scientific research as a simple model for the study of biological processes that occur in larger, more complex animals — including humans.
The round worm is Gallegos’ research vehicle of choice, and the professor says there are still plenty of questions surrounding its nervous system development that she is interested in tackling — and that CRISPR promises to accelerate the discovery of.
“How neurons create and maintain neural circuits is critical to behavior,” Gallegos explains. “For instance, there is a protein (Unc-11) we study that disrupts neuron development and was first described in C. elegans. Its counterpart in humans has more recently been linked to an increased risk of Alzheimer’s.”
“Since no one else has done this before, I knew it must be hard. There must be something tricky about it. And if it’s hard, I want to try it.”
Last year, a student in Gallegos’ class created the tools needed to perform a knock-in at the Unc-11 site, adding a green fluorescent protein to the round worm’s genome so that the lab could follow where the protein travels inside the worm. Importantly, because the worm’s genome inside a fertilized egg was successfully modified, its descendants also carry the fluorescent protein and they too can be used in future research.
It’s the type of work that Gallegos wants to do on another protein in the round worm that she has long been interested in, one called Sax-2. Scientists know it plays a role in neurodevelopment, but older methods of attempting to trace its journey in the development of the worm have proven error-prone.
That alone was enough to inspire master’s candidate Ramon Benito to accept the challenge of fusing a fluorescent tracer to the mysterious protein using CRISPR — a task Gallegos says hasn’t been done before and could lead to new and/or more precise information about when, where and how it functions. It’s just one of several projects students in her class picked from to gain firsthand exposure to the CRISPR technique.
“Since no one else has done this before, I knew it must be hard,” Benito says. “There must be something tricky about it. And if it’s hard, I want to try it.”
Benito, a first-generation student who hopes to someday work in virology and immunology, says it’s the type of big-impact experience that has inspired him to become a researcher rather than a doctor.
“I want to help as many people as possible,” he says. “And if I’m a doctor, I can only see so many patients. But as a research scientist, if you can find something novel that can lead to a new drug, it could impact thousands of people.”
NEW BEGINNINGS
With Gallegos’ supervision, Benito has successfully created the molecular tools needed to fuse the green fluorescent protein to Sax-2 in the round worm.
“The DNA will now have to be injected into the worm’s gonad with a microscopic glass needle,” Gallegos explains. “With just a small bit of luck, Cas9 will cut the worm’s genome at the right place and the organism will go to work repairing the cut with the matching DNA. In this way, the gene that produces the green fluorescent protein will be inserted into the exact site of where Sax-2 is located in the worm, and hopefully we can learn more about its function.”
“I want to be on the forefront of discovery, and Cal State East Bay is helping me to do that.”
Since the quarter ended, Gallegos has been verifying Benito and other students’ work, in preparation for injecting the DNA into the worms. Her ultimate goal is to publish alongside the students.
“What’s interesting for me is that the same genes required to build this worm’s simple nervous system have been shown to function in the neurodevelopment of other organisms, including humans,” she says. “Which means if we can not only learn how these genes work during normal development, but also in injury and disease, perhaps we can harness that information to help people recover from injury or disease.”
For Benito, it’s promise enough to push him toward applying to obtain his Ph.D. once he finishes two separate master’s theses at Cal State East Bay next year — and continue using CRISPR.
“It’s important to remember that CRISPR is only a way to cut DNA,” he says. “It’s the secondary technique, the knock in, that creates the endless possibilities. That’s where the engineering happens. We don’t know if Sax-2 has any relevance to disease, but if it does, this is the first step to finding out. I want to be on the forefront of discovery, and Cal State East Bay is helping me to do that.”
THE BATTLE FOR CRISPR: PATENT FIGHTS, THE BIOTECH INDUSTRY AND HUSH-HUSH DRUG DEVELOPMENT
When scientific scholars Jennifer Doudna (UC Berkeley) and Emmanuelle Charpentier (then at Umeå University in Sweden) published their groundbreaking 2012 paper on a CRISPR/Cas9 gene-editing technique, they concluded the manuscript with this promising line: “We propose an alternative methodology based on RNA-programmed Cas9 that could offer considerable potential for gene-targeting and genome-editing applications.” For non-science persons, what the researchers were really saying was something like this: “This paper pertains to bacteria in test tubes, but hold tight, we’ll be right back with evidence it works on DNA inside a cell, plant or animal of choice.”
The trouble is, as often happens with a new, revolutionary technique, a researcher at Harvard’s Broad Institute named Feng Zhang was busy taking the same idea about CRISPR/Cas9 one step further by jumping directly to cutting DNA in human cells, a feat he published in 2013. Anticipating Zhang’s success, the Broad Institute filed a dozen expedited applications with the U.S. Patent and Trademark Office in 2012. Before Doudna and Charpentier could fast-track their own patents, the Broad Institute was awarded ownership of using CRISPR to edit genomes or other sequences of DNA for a variety of purposes.
Shortly thereafter, those patents (now in the appeals process) were challenged by the UC/Doudna team, who contend their watershed publication is deserving of any and all patents on CRISPR. “They have a patent on green tennis balls. We [likely] will have a patent on all tennis balls,” Doudna has said.
In the meantime, biotech labs across the country are already using CRISPR, including those with Cal State East Bay students working in them. One master’s student who wished to remain anonymous said, “I use absolutely every skill set I learned at the university through the master’s program and I work in CRISPR every day — the company I work for is trying to develop a drug based on CRISPR technology, and they’ve requested that I not advertise what I’m doing or where I work.” When asked about the need for secrecy, Gallegos explained that while some companies are openly using CRISPR and have taken a “let the chips fall where they may” attitude toward the patent dispute (given its potential to help human life now), “others are likely trying to keep quiet about their techniques for fear that whoever ultimately wins can come after them for whatever technologies or drugs have been developed by that time.”
Still, Gallegos isn’t concerned about the implications for her classroom. Both Doudna and Feng have pledged to release all intellectual property to academic researchers for free — and Gallegos has plenty of work in her pipeline. “I will never run out of novel projects for my students,” she says. “There are more than 20,000 genes in the worm genome.”